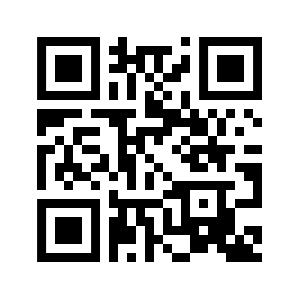
Efficient Room Temperature Ethanol Vapor Sensing by Unique Fractal Features of Tin Oxide
Materials Science SensorsReceived 09 Jan 2024 Accepted 09 Feb 2024 Published online 12 Feb 2024
Focusing on Biology, Medicine and Engineering ISSN: 2995-8067 | Quick Google Scholar
Next Full Text
The Kazakh Language Requires Reform of its Writing
Previous Full Text
Strengthening IoT Network Protocols: A Model Resilient Against Cyber Attacks
Received 09 Jan 2024 Accepted 09 Feb 2024 Published online 12 Feb 2024
Fractals are complex structures that repeat themselves at several scales. Nature exhibits these in many forms like snowflakes, mountains, coastlines, the human brain/lungs/ nervous system, and many more. It appears that these are nature’s organic way of growth. Thus, there is an underlying science that works to grow or create these self-similar patterns. In this work, tin oxide-based fractals have been grown under laboratory conditions and applied to a gas-sensing field. The facile growth methodology successfully grows fractals on a large scale. The tin oxide fractals have unique basic building units that connect and grow in different directions. These tin oxide fractals have successfully sensed ethanol vapors in the range of 20 ppm to 100 ppm. The best sensing response has also detected ethanol vapors as low as 10 ppm at room temperature with response and recovery times of 18 ± 3 s and 22 ± 5 s, respectively. The best sensing response recorded for such sensors was under 12 s. The characteristic fractal growth is attributed as the defining factor that enhances ethanol sensing at room temperature.
As digital technologies become more widespread research on sensors and device communication in the automation field needs to be given due attention. Highly sensitive sensors that can adapt to different environmental conditions are necessary for effective device interaction. In order to progress in the digital age, sensors and device communication systems must work together. Figure 1 depicts the US sensor market’s over-year growth in demand for sensors that can detect different gases [
]. Ethanol sensors are used in various industries like chemical, food, ethanol production, and breweries [ - ]. It is critical to have an efficient sensor capable of operating at room temperature, as sensors operating at higher temperatures consume more power. Many metal oxides, including Sn, Zn, Ti, and others, have found application as sensors [ - ]. However, easy synthesis methodology along with good sensing behavior is always not easy to address. Researchers frequently use techniques like doping, annealing, dispersing nanoparticles, etc. to improve sensor performance [ - ]. Morphology is one of the crucial factors in semiconductor oxide gas sensor study since it provides a platform for the adsorption of gas analytes and deciding the rate of reaction to take place on the sensing surface. Sensing surfaces with significant morphology greatly improves gas-sensing abilities [ , ].In this work tin oxide fractals with various morphologies were obtained by the sol-gel assisted method followed by the microwave irradiation technique. The precipitate thus obtained was dried under a hot air oven. The dried sample showed fascinating fractal morphologies at macro scales with branching structures. The prepared fractal morphology exhibited efficient ethanol vapor sensing detection ability without applying any heat treatment to the prepared sensor.
To obtain the fractal-like morphologies, chemicals such as tin chloride pentahydrate (SnCl4.5H2O, 98% purity) and Urea (H2NCONH2, 99% purity) were received from Molychem (India) and Fisher Scientific (India), respectively and used further without any purification. Distilled water was used throughout for synthesis purposes. Briefly, sols were prepared in aqueous media using tin chloride pentahydrate and urea, the details can be found elsewhere [
]. No heating was applied at any stage. The reaction mixture was irradiated by microwaves at 595 watts. The reaction mixture turned turbid after 8 minutes and was allowed to cool naturally. Thereafter, it was dried in a hot air oven at a moderate temperature of 70 °C until completely dry. When fractals of different sizes and shapes emerge. The white precipitate formed was crushed manually using an agate mortar and pestle and stored in clean and dry conditions under a vacuum until further use.X-ray diffraction (XRD) was used to examine the sample’s phase formation and crystalline structure by using a Rigaku, Miniflex-600 instrument with Cu-Kα radiation at a wavelength of 1.5418 Å. The functional groups present in the sample were investigated using Shimadzu IR Affinity 1 FTIR spectroscopy. A gas-sensing apparatus by Prism Electronics Systems, India with 5V dc bias voltage was used for investigating the gas-sensing characteristics of the samples at room temperature. The response of the sensor was measured by changing the electrical resistance of the sensor with respect to the specific concentration of ethanol vapor exposed to the sensor at room temperature.
In this work, fractal morphology forms as a result of precisely calibrated heating and salt concentration. The sol-gel-assisted method, followed by microwave irradiation, provides an easy and inexpensive method to synthesize the material. The fractal morphology was reported for other oxide materials by various synthesis techniques such as chemical vapor deposition, hydrothermal, sol-gel, microwave, pulse vapor deposition spray pyrolysis, etc [
- ]. In order to form fractal morphologies of various materials, it is difficult to optimize temperature and synthesis parameters. Furthermore, accurately determining the fractal characteristics of various morphological fractal structures is sometimes difficult. The fractal morphology of the tin oxide material obtained in this work is simple and at a lower temperature when compared to other published work. Figure 2(a,b) shows the tin oxide fractals grown on a glass substrate with very fine dendritic structures. The XRD analysis revealed the presence of impurities in the as-synthesized sample. Whereas, diffraction peaks at 26.6°, 33.8°, 37.8°, 51.6°, 54.2°, 57.8°, 62, 65°, 71.3° and 78.7° corresponded to the (110), (101), (200), (211), (220), (002), (310), (112), (202) and (321) crystal faces of tetragonal SnO2 structure respectively for annealed sample at 400 °C. All the diffraction peaks match well with JCPDS card number 041-1445 of SnO2 [ , ]. There were no impurities and characteristic peaks of other types of tin oxide found. Figure 2(c) shows that the X-ray diffractogram collected on the sample annealed at 400 °C in air and inset with the X-ray diffractogram of the as-synthesized fractal sample. The crystallite size of the as-synthesized and annealed sample was calculated to be 45 ± 5 nm and 10 ± 2 nm respectively by using Scherrer’s equation [ ]. Whereas, according to the Williamson-Hall (W-H) model size and strain broadening have been obtained by considering peak width as a function of 2θ [ , ]. The W-H model resulted in a crystallite size of maximum intense peak of the annealed sample to be 9.2 nm and a strain value be 0.2%.The FTIR spectrum shown in Figure 2(d) was analyzed to identify the structural information of functional groups present on the surface of the sample. The absorption peaks at 535 cm-1 and 866 cm-1 indicate O-Sn-O and Sn-OH stretching vibration mode, respectively. The peaks at 1465 cm−1 are attributed to stretching vibrations of the O-H bond [
, ]. Weak peaks detected around 2300 cm-1 are most likely due to water or CO2 being absorbed from the surrounding atmosphere.Numerous studies have looked into the performance of fractal-based gas sensors. Some studies concentrate on the fractal morphologies of sensing materials at different temperatures, while others look into the fractal-shaped structure of the sensing substrate with varying dimensions [
- ]. The ultimate goal of this investigation is to improve the material’s sensing performance. In this work, to study the sensing performance of the fractal sample, the homogenous slurry of the fractal material was coated uniformly over the glass substrate to form a thick film. Iso-propanol was used as a solvent for slurry preparation. Electrical contact pads were made on the glass substrate using highly conducting silver epoxy. The sensor was connected to the in-house developed gas sensing set-up. The sensor was exposed to ethanol vapors with 20 to 100 ppm concentration. The sensor was biased at 5V and all the testing was conducted at room temperature. Figure 3 shows the sensing response of the sensor for 20-100 ppm of ethanol concentration with (a) a change in resistance and (b) normalized percentage response values. The fast-sensing response is attributed to the unique dendritic morphology of fractals which offer cross-connectivity between fractal fingers providing an electronically connected network of the sensing material, surface roughness, and abundant adsorption sites for interaction with target gas analytes [ , ]. The senor showed an average response time of ~18 ± 3s and an average recovery time of ~22 ± 5s at room temperature. In light of the foregoing, it is concluded that fractal morphology prepared using SnO2 material at moderate conditions plays an important role in the sensing field because nano/micro scale fractal materials provide high porosity and surface area, high physical connectivity within patterned objects, and are an innovative way to improve sensing performance of known materials.In summary, the sol-gel and microwave irradiation methods were used to obtain fractals with crystallite size of 45 ± 5 nm (as-synthesized) and 12 ± 2 nm (for annealed samples) as estimated from Scherrer’s equation. The material successfully detected ethanol vapors in the range of 20-100 ppm concentration. The resulting material exhibited an average response time of ~18 ± 3s and average recovery time of ~22 ± 5s.
The gas sensing field is an active area of research. Whereas the sensor performance on substrates designed as fractals has been reported, reports on fractal material as sensing material are very few. This work demonstrates an innovative and facile synthesis methodology to mimic the nature and grow fractals of tin oxide. The material shows a great performance in sensing ethanol. This work highlights that apart from doping, band-structure engineering, nano-sizing, and raising the temperature, there is yet another unique way to enhance sensing performance by using fractals.
Author VK would like Symbiosis Centre for Research and Innovations (SCRI) International (Deemed University) for a Senior Research Fellowship. The authors acknowledge the Symbiosis International (Deemed University) for the Research Support Fund.
Gas Sensor Market Size & Share Analysis. 2023; 113.
Konstantaki M, Klini A, Anglos D, Pissadakis S. An ethanol vapor detection probe based on a ZnO nanorod coated optical fiber long period grating. Opt Express. 2012 Apr 9;20(8):8472-84. doi: 10.1364/OE.20.008472. PMID: 22513555.
Li B, Zhou Q, Peng S, Liao Y. Recent Advances of SnO2-Based Sensors for Detecting Volatile Organic Compounds. Front Chem. 2020 May 5;8:321. doi: 10.3389/fchem.2020.00321. PMID: 32432077; PMCID: PMC7214870.
Memon SF, Wang R, Strunz B, Chowdhry BS, Pembroke JT, Lewis E. A Review of Optical Fibre Ethanol Sensors: Current State and Future Prospects. Sensors (Basel). 2022 Jan 26;22(3):950. doi: 10.3390/s22030950. PMID: 35161695; PMCID: PMC8840036.
Huang Z,Wang W, Xiao D, Mo Y, Wang S, Xu Z. Ethanol gas sensing with lower temperature and higher response based on three-dimensional multilevel materials composed of micron hollow carbon spheres@SnO2 nanoparticles, Materials Today Communications. 2022; 32: 104105.
Kang Y, Yu F, Zhang L, Wang W, Chen L, Li Y. Review of ZnO-based nanomaterials in gas sensors, Solid State Ionics. 2021; 360: 115544.
Li Z, Yao Z, Haidry AA, Plecenik T, Xie L, Sun L. Resistive-type hydrogen gas sensor based on TiO2: A review. International Journal of Hydrogen Energy. 2018; 43: 21114-32.
Haidry AA, Yucheng W, Fatima Q, Raza A, Zhong L, Chen H. Synthesis and characterization of TiO2 nanomaterials for sensing environmental volatile compounds (VOCs): A review. TrAC Trends in Analytical Chemistry. 2023; 117454.
Paul D, Aamir L, Aslam A, Rathore D. p-/n-Type Switching in the Ag/BTO/TiO2Nanocomposite as a Gas Sensor toward Ethanol, Liquefied Petroleum Gas, and Ammonia. Langmuir. 2023 Aug 22;39(33):11879-11887. doi: 10.1021/acs.langmuir.3c01687. Epub 2023 Aug 10. PMID: 37562969.
Khosravani S, Hajakbari F, Hojabri A. Preparation, characterization and gas sensor performance of nanocrystalline nickel-doped SnO2 films. Journal of Materials Science: Materials in Electronics. 2023; 34: 1515.
Kornyushchenko A, Kosminska Y, Stas S, Wilde G, Perekrestov V. Structural, Morphological and Sensor Properties of the Fractal-Percolation Nanosystem ZnO/NiO. Journal of Electronic Materials. 2021; 50: 2268-76.
Rodrigues J, Jain S, Shimpi NG. Performance of 1D tin (Sn) decorated spherical shape ZnO nanostructures as an acetone gas sensor for room and high temperature. Materials Science and Engineering: B. 2023; 288:116199.
Tharsika T, Thanihaichelvan M, Haseeb A, Akbar SA. Highly sensitive and selective ethanol sensor based on ZnO nanorod on SnO2 thin film fabricated by spray pyrolysis. Frontiers in Materials. 2019; 6: 122.
Li YX, Guo Z, Su Y, Jin XB, Tang XH, Huang JR, Huang XJ, Li MQ, Liu JH. Hierarchical Morphology-Dependent Gas-Sensing Performances of Three-Dimensional SnO2 ACS Sens. 2017 Jan 27;2(1):102-110. doi: 10.1021/acssensors.6b00597. Epub 2016 Dec 20. PMID: 28722446.
Lin T, Lv X, Li S, Wang Q. The Morphologies of the Semiconductor Oxides and Their Gas-Sensing Properties. Sensors (Basel). 2017 Nov 30;17(12):2779. doi: 10.3390/s17122779. PMID: 29189714; PMCID: PMC5751450.
Kamathe V, Nagar R. Large-scale growth of tin oxide fabricated fractals. Journal of Sol-Gel Science and Technology. 2022; 101: 477-83.
Chen Z, Pan D, Zhao B, Ding G, Jiao Z, Wu M, Shek CH, Wu LC, Lai JK. Insight on fractal assessment strategies for tin dioxide thin films. ACS Nano. 2010 Feb 23;4(2):1202-8. doi: 10.1021/nn901635f. PMID: 20085367.
Fusco Z, Rahmani M, Bo R, Verre R, Motta N, Käll M, Neshev D, Tricoli A. Nanostructured Dielectric Fractals on Resonant Plasmonic Metasurfaces for Selective and Sensitive Optical Sensing of Volatile Compounds. Adv Mater. 2018 Jul;30(30):e1800931. doi: 10.1002/adma.201800931. Epub 2018 Jun 4. PMID: 29862583.
Sabri YM, Kandjani AE, Rashid SSAAH, Harrison CJ, Ippolito SJ, Bhargava SK. Soot template TiO2 fractals as a photoactive gas sensor for acetone detection. Sensors and Actuators B: Chemical. 2018; 275: 215-22.
Guo J, Zhang J, Gong H, Ju D, Cao B. Au nanoparticle-functionalized 3D SnO2 microstructures for high performance gas sensor. Sensors and Actuators B: Chemical. 2016; 226: 266-72.
Kopnov G, Das SS, Gerber A. Effect of Fractal Topology on the Resistivity Response of Thin Film Sensors. Sensors (Basel). 2023 Feb 22;23(5):2409. doi: 10.3390/s23052409. PMID: 36904619; PMCID: PMC10007381.
Tran-Phu T, Daiyan R, Fusco Z, Ma Z, Abd Rahim LR, Kiy A. Multifunctional nanostructures of Au–Bi 2 O 3 fractals for CO 2 reduction and optical sensing. Journal of Materials Chemistry A. 2020; 8: 11233-45.
Abdelkrim A, Rahmane S, Abdelouahab O, Abdelmalek N, Brahim G. Effect of solution concentration on the structural. Optical and electrical properties of SnO2 thin films prepared by spray pyrolysis. Optik. 2016; 127: 2653-8.
Kadhim IH, Hassan HA, Ibrahim FT. Hydrogen gas sensing based on nanocrystalline SnO2 thin films operating at low temperatures. International Journal of Hydrogen Energy. 2020; 45: 25599-607.
Bokuniaeva AO, Vorokh AS. Estimation of particle size using the Debye equation and the Scherrer formula for polyphasic TiO2 powder, Journal of Physics: Conference Series.2019; 1410: 012057.
Kim YY, Schenk AS, Ihli J, Kulak AN, Hetherington NB, Tang CC, Schmahl WW, Griesshaber E, Hyett G, Meldrum FC. A critical analysis of calcium carbonate mesocrystals. Nat Commun. 2014 Jul 11;5:4341. doi: 10.1038/ncomms5341. PMID: 25014563; PMCID: PMC4104461.
Nath D, Singh F, Das R. X-ray diffraction analysis by Williamson-Hall, Halder-Wagner and size-strain plot methods of CdSe nanoparticles- a comparative study, Materials Chemistry and Physics. 2020; 239: 122021.
Akkera HS, Mann V, Varalakshmi BN, Ploloju M, Kambhala N, Venkatesh G. Effect of Sr-doped on physical and photoluminescence properties of SnO2 transparent conducting oxide thin films. Journal of Materials Science: Materials in Electronics. 2023; 34:1044.
Amalric-Popescu D, Bozon-Verduraz F. Infrared studies on SnO2 and Pd/SnO2. Catalysis Today. 2001; 70:139-54.
Fusco Z, Rahmani M, Tran-Phu T, Ricci C, Kiy A, Kluth P, Della Gaspera E, Motta N, Neshev D, Tricoli A. Photonic Fractal Metamaterials: A Metal-Semiconductor Platform with Enhanced Volatile-Compound Sensing Performance. Adv Mater. 2020 Dec;32(50):e2002471. doi: 10.1002/adma.202002471. Epub 2020 Oct 22. PMID: 33089556.
Yang T. Optimizing electrode structure of carbon nanotube gas sensors for sensitivity improvement based on electric field enhancement effect of fractal geometry. Scientific Reports. 2021; 11:16675.
Yang T, Tian F, Covington JA, Xu F, Xu Y, Jiang A. Resistance-capacitance gas sensor based on fractal geometry. Chemosensors. 2019; 7: 31.
Jiang A, Tian F, Covington JA, Jiang M, Wu Z. Development of gas sensor based on fractal substrate structures. IEEE Transactions on Instrumentation and Measurement. 2022; 71:1-7.
Kamathe V, Nagar R. Morphology-driven gas sensing by fabricated fractals: A review. Beilstein Journal of Nanotechnology. 2021; 12:1187-208.
Tian F, Jiang A, Yang T, Qian J, Liu R, Jiang M. Application of fractal geometry in gas sensor: A review. IEEE Sensors Journal. 2021; 21:14587-600.
Nagar R, Kamathe V. Efficient Room Temperature Ethanol Vapor Sensing by Unique Fractal Features of Tin Oxide. IgMin Res. 12 Feb, 2024; 2(2): 097-101. IgMin ID: igmin150; DOI: 10.61927/igmin150; Available at: www.igminresearch.com/articles/pdf/igmin150.pdf
Anyone you share the following link with will be able to read this content:
Address Correspondence:
Rupali Nagar, Nanomaterials for Energy Applications Lab, Applied Science Department, Symbiosis Institute of Technology, Symbiosis International (Deemed University), Lavale, Pune-412115, Maharashtra, India, Email: [email protected]
How to cite this article:
Nagar R, Kamathe V. Efficient Room Temperature Ethanol Vapor Sensing by Unique Fractal Features of Tin Oxide. IgMin Res. 12 Feb, 2024; 2(2): 097-101. IgMin ID: igmin150; DOI: 10.61927/igmin150; Available at: www.igminresearch.com/articles/pdf/igmin150.pdf
Copyright: © 2024 Nagar R, et al. This is an open access article distributed under the Creative Commons Attribution License, which permits unrestricted use, distribution, and reproduction in any medium, provided the original work is properly cited.
Gas Sensor Market Size & Share Analysis. 2023; 113.
Konstantaki M, Klini A, Anglos D, Pissadakis S. An ethanol vapor detection probe based on a ZnO nanorod coated optical fiber long period grating. Opt Express. 2012 Apr 9;20(8):8472-84. doi: 10.1364/OE.20.008472. PMID: 22513555.
Li B, Zhou Q, Peng S, Liao Y. Recent Advances of SnO2-Based Sensors for Detecting Volatile Organic Compounds. Front Chem. 2020 May 5;8:321. doi: 10.3389/fchem.2020.00321. PMID: 32432077; PMCID: PMC7214870.
Memon SF, Wang R, Strunz B, Chowdhry BS, Pembroke JT, Lewis E. A Review of Optical Fibre Ethanol Sensors: Current State and Future Prospects. Sensors (Basel). 2022 Jan 26;22(3):950. doi: 10.3390/s22030950. PMID: 35161695; PMCID: PMC8840036.
Huang Z,Wang W, Xiao D, Mo Y, Wang S, Xu Z. Ethanol gas sensing with lower temperature and higher response based on three-dimensional multilevel materials composed of micron hollow carbon spheres@SnO2 nanoparticles, Materials Today Communications. 2022; 32: 104105.
Kang Y, Yu F, Zhang L, Wang W, Chen L, Li Y. Review of ZnO-based nanomaterials in gas sensors, Solid State Ionics. 2021; 360: 115544.
Li Z, Yao Z, Haidry AA, Plecenik T, Xie L, Sun L. Resistive-type hydrogen gas sensor based on TiO2: A review. International Journal of Hydrogen Energy. 2018; 43: 21114-32.
Haidry AA, Yucheng W, Fatima Q, Raza A, Zhong L, Chen H. Synthesis and characterization of TiO2 nanomaterials for sensing environmental volatile compounds (VOCs): A review. TrAC Trends in Analytical Chemistry. 2023; 117454.
Paul D, Aamir L, Aslam A, Rathore D. p-/n-Type Switching in the Ag/BTO/TiO2Nanocomposite as a Gas Sensor toward Ethanol, Liquefied Petroleum Gas, and Ammonia. Langmuir. 2023 Aug 22;39(33):11879-11887. doi: 10.1021/acs.langmuir.3c01687. Epub 2023 Aug 10. PMID: 37562969.
Khosravani S, Hajakbari F, Hojabri A. Preparation, characterization and gas sensor performance of nanocrystalline nickel-doped SnO2 films. Journal of Materials Science: Materials in Electronics. 2023; 34: 1515.
Kornyushchenko A, Kosminska Y, Stas S, Wilde G, Perekrestov V. Structural, Morphological and Sensor Properties of the Fractal-Percolation Nanosystem ZnO/NiO. Journal of Electronic Materials. 2021; 50: 2268-76.
Rodrigues J, Jain S, Shimpi NG. Performance of 1D tin (Sn) decorated spherical shape ZnO nanostructures as an acetone gas sensor for room and high temperature. Materials Science and Engineering: B. 2023; 288:116199.
Tharsika T, Thanihaichelvan M, Haseeb A, Akbar SA. Highly sensitive and selective ethanol sensor based on ZnO nanorod on SnO2 thin film fabricated by spray pyrolysis. Frontiers in Materials. 2019; 6: 122.
Li YX, Guo Z, Su Y, Jin XB, Tang XH, Huang JR, Huang XJ, Li MQ, Liu JH. Hierarchical Morphology-Dependent Gas-Sensing Performances of Three-Dimensional SnO2 ACS Sens. 2017 Jan 27;2(1):102-110. doi: 10.1021/acssensors.6b00597. Epub 2016 Dec 20. PMID: 28722446.
Lin T, Lv X, Li S, Wang Q. The Morphologies of the Semiconductor Oxides and Their Gas-Sensing Properties. Sensors (Basel). 2017 Nov 30;17(12):2779. doi: 10.3390/s17122779. PMID: 29189714; PMCID: PMC5751450.
Kamathe V, Nagar R. Large-scale growth of tin oxide fabricated fractals. Journal of Sol-Gel Science and Technology. 2022; 101: 477-83.
Chen Z, Pan D, Zhao B, Ding G, Jiao Z, Wu M, Shek CH, Wu LC, Lai JK. Insight on fractal assessment strategies for tin dioxide thin films. ACS Nano. 2010 Feb 23;4(2):1202-8. doi: 10.1021/nn901635f. PMID: 20085367.
Fusco Z, Rahmani M, Bo R, Verre R, Motta N, Käll M, Neshev D, Tricoli A. Nanostructured Dielectric Fractals on Resonant Plasmonic Metasurfaces for Selective and Sensitive Optical Sensing of Volatile Compounds. Adv Mater. 2018 Jul;30(30):e1800931. doi: 10.1002/adma.201800931. Epub 2018 Jun 4. PMID: 29862583.
Sabri YM, Kandjani AE, Rashid SSAAH, Harrison CJ, Ippolito SJ, Bhargava SK. Soot template TiO2 fractals as a photoactive gas sensor for acetone detection. Sensors and Actuators B: Chemical. 2018; 275: 215-22.
Guo J, Zhang J, Gong H, Ju D, Cao B. Au nanoparticle-functionalized 3D SnO2 microstructures for high performance gas sensor. Sensors and Actuators B: Chemical. 2016; 226: 266-72.
Kopnov G, Das SS, Gerber A. Effect of Fractal Topology on the Resistivity Response of Thin Film Sensors. Sensors (Basel). 2023 Feb 22;23(5):2409. doi: 10.3390/s23052409. PMID: 36904619; PMCID: PMC10007381.
Tran-Phu T, Daiyan R, Fusco Z, Ma Z, Abd Rahim LR, Kiy A. Multifunctional nanostructures of Au–Bi 2 O 3 fractals for CO 2 reduction and optical sensing. Journal of Materials Chemistry A. 2020; 8: 11233-45.
Abdelkrim A, Rahmane S, Abdelouahab O, Abdelmalek N, Brahim G. Effect of solution concentration on the structural. Optical and electrical properties of SnO2 thin films prepared by spray pyrolysis. Optik. 2016; 127: 2653-8.
Kadhim IH, Hassan HA, Ibrahim FT. Hydrogen gas sensing based on nanocrystalline SnO2 thin films operating at low temperatures. International Journal of Hydrogen Energy. 2020; 45: 25599-607.
Bokuniaeva AO, Vorokh AS. Estimation of particle size using the Debye equation and the Scherrer formula for polyphasic TiO2 powder, Journal of Physics: Conference Series.2019; 1410: 012057.
Kim YY, Schenk AS, Ihli J, Kulak AN, Hetherington NB, Tang CC, Schmahl WW, Griesshaber E, Hyett G, Meldrum FC. A critical analysis of calcium carbonate mesocrystals. Nat Commun. 2014 Jul 11;5:4341. doi: 10.1038/ncomms5341. PMID: 25014563; PMCID: PMC4104461.
Nath D, Singh F, Das R. X-ray diffraction analysis by Williamson-Hall, Halder-Wagner and size-strain plot methods of CdSe nanoparticles- a comparative study, Materials Chemistry and Physics. 2020; 239: 122021.
Akkera HS, Mann V, Varalakshmi BN, Ploloju M, Kambhala N, Venkatesh G. Effect of Sr-doped on physical and photoluminescence properties of SnO2 transparent conducting oxide thin films. Journal of Materials Science: Materials in Electronics. 2023; 34:1044.
Amalric-Popescu D, Bozon-Verduraz F. Infrared studies on SnO2 and Pd/SnO2. Catalysis Today. 2001; 70:139-54.
Fusco Z, Rahmani M, Tran-Phu T, Ricci C, Kiy A, Kluth P, Della Gaspera E, Motta N, Neshev D, Tricoli A. Photonic Fractal Metamaterials: A Metal-Semiconductor Platform with Enhanced Volatile-Compound Sensing Performance. Adv Mater. 2020 Dec;32(50):e2002471. doi: 10.1002/adma.202002471. Epub 2020 Oct 22. PMID: 33089556.
Yang T. Optimizing electrode structure of carbon nanotube gas sensors for sensitivity improvement based on electric field enhancement effect of fractal geometry. Scientific Reports. 2021; 11:16675.
Yang T, Tian F, Covington JA, Xu F, Xu Y, Jiang A. Resistance-capacitance gas sensor based on fractal geometry. Chemosensors. 2019; 7: 31.
Jiang A, Tian F, Covington JA, Jiang M, Wu Z. Development of gas sensor based on fractal substrate structures. IEEE Transactions on Instrumentation and Measurement. 2022; 71:1-7.
Kamathe V, Nagar R. Morphology-driven gas sensing by fabricated fractals: A review. Beilstein Journal of Nanotechnology. 2021; 12:1187-208.
Tian F, Jiang A, Yang T, Qian J, Liu R, Jiang M. Application of fractal geometry in gas sensor: A review. IEEE Sensors Journal. 2021; 21:14587-600.