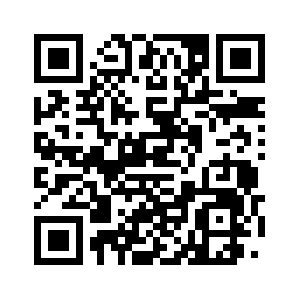
Investigation and Energy Modeling of New Generation Environmentally Friendly Energy Source Thorium Fueled Molten Salt Reactors
Mechanical EngineeringReceived 04 Dec 2024 Accepted 22 Apr 2025 Published online 24 Apr 2025
ISSN: 2995-8067 | Quick Google Scholar
Received 04 Dec 2024 Accepted 22 Apr 2025 Published online 24 Apr 2025
A new generation of innovative nuclear reactor technology is being piloted in some countries. This reactor features low installation and electricity production costs, a much-reduced waste problem, and a simplified yet reliable nuclear fuel cycle. It is designed for long-term energy production—at least 60 years—while utilizing domestic resources to significantly reduce costs, including those related to fuel raw material and manufacturing. The thorium fuel cycle molten salt reactor is at the heart of this advancement, offering a promising solution for sustainable nuclear energy. In thorium MSRs (Molten Salt Reactors), the giant pressure vessel, fuel bundles and reactor heart, complex piping systems and pumps that are in conventional reactors of the same power are either completely absent or have been reduced much more. For example, the main body of a 1500 MWe MSR is the size of 2 truck containers and occupies relatively little space. There is no accidental hydrogen release or reactor explosion in MSRs due to their design. Since the design minimizes explosion risk, the likelihood of uncontrolled radioactive release is significantly reduced of radioactive isotopes into the atmosphere, water or soil. In case of a problem during operation, there is no need for human intervention to stop the system. MSR fuels are molten at 600 °C - 700 °C; the hearth where they are located is not at very high pressure, but at normal pressure, there is no solid fuel rods or water in the environment, so the risk of explosion could be refined to reflect that risks are minimized (not eliminated). In the unlikely event of overheating, liquid fuel flows from the discharge pipe at the bottom to the liquid fuel tank, the nuclear reaction stops and these processes occur without human intervention. MSRs do not require human intervention during regular operation either. Because the system is designed to operate continuously and safely on its own. The radioactive waste from MSRs is much less in volume and their storage life is only 300 years, not 10s of thousands of years, which makes it easier in this respect. In this study, the advantages, disadvantages, and energy effects of the new-generation energy source—thorium-fueled molten salt reactors—were examined, with explanations provided on their contributions and energy modeling.
Molten Salt Reactors (MSRs) demonstrate a promising technology in the nuclear energy area, notwithstanding their relatively long history. When examining the historical development of MSRs;
When we go back to the 1940s, as nuclear energy was being explored, diversified coolant and fuel types were investigated. Molten salts were regarded as promising coolants because of their ability to withstand high temperatures and their properties for moderating neutrons [
].When it comes to the 1950s, the Oak Ridge National Laboratory (ORNL) in the United States governed the first tests on molten salt reactors. During these tests, molten salt served a dual role as both a coolant and a fuel [
].In the 1960s, ORNL initiated a more spacious molten salt reactor initiative known as the Molten-Salt Reactor Experiment (MSRE). Lasting for approximately, the MSRE demonstrated that molten salt reactors could be convenient. Nevertheless, the project was ultimately discontinued for diversified reasons [
].The period between 1970 and 1990, the excitement for developing technologies like molten salt reactors diminished due to oil crises and increasing concerns about nuclear energy.
Considering the technological developments of the 2000s, as fears about global warming and the finited supply of fossil fuels intensified, interest in nuclear power re-emerged. The search for safer and more environmentally friendly nuclear reactor technologies brought molten salt reactors back into the spotlight [ ].
Numerous countries and corporations are investigating molten salt reactors, considering the level of development of today's conditions. In particular, thorium-fueled molten salt reactors are viewed as a promising alternative for generating nuclear energy that is more sustainable and safer [
].MSRs are another promising technology in the nuclear energy field. These reactors utilize thorium as their fuel and molten salts for cooling, opening up new possibilities for generating nuclear energy [
] (Figure 1).Explanations of the pieces in the picture:
Tire particle inlet: Input where the tire particles that will enter the pyrolysis process are taken into the system.
Molten salt inlet/outlet: Points where the molten salt enters and exits the reactor; Used for heat transfer.
Molten salt tank: Tank where molten salt is stored and prepared for circulation in the system.
Molten salt pump: Pump that circulates the molten salt in the system.
Temperative control system: Unit that monitors and controls the temperature of the system.
Gas outlet: The point where the gases formed as a result of pyrolysis come out of the system.
Condensation system: The system in which pyrolysis gases are concentrated on the oil form.
Pyrolysis gas: The gas produced by pyrolysis process indicated by the yellow line.
Pyrolysis char tank: The tank where the solid coal formed as a result of pyrolysis is collected.
Pyrolysis oil tank: Tank where condensed pyrolysis oil is stored with a red line.
Gas tank: Tank where the remaining gases are stored after condensation.
Char outlet: The starting point where pyrolysis coal is taken from the system.
Molten salt fuel: The fuel is held within a molten salt mixture. This way, the fuel moves continuously and ensures an even spread within the reactor [
].Fast neutron spectrum: Fast neutrons are utilized in the reactor. This contributes to reducing nuclear waste generation and creating a more effective fuel cycle [
].Thorium fuel: MSRs typically utilize thorium fuel. Thorium is a more plentiful element compared to uranium and possesses a high capability for nuclear energy generation.
Passive safety: The arrangement of the reactor is focused on guaranteeing safety by relying on natural physical mechanisms. This design significantly reduces the likelihood of nuclear accidents.
Many research centers and academic institutions in Europe are carrying out research on MSR technology. The emphasis of these studies is on enhancing MSRs regarding safety, efficiency, and environmental responsibility [
].France: France is among the leading nations in MSR technology (CEA Reports, 2022). French scientists are performing detailed studies to find out the essential design factors of MSRs and to carry out safety evaluations.
Germany: Germany has also made notable advances in MSR technology. German scientists are especially concentrating on the issues of nuclear waste management and safety related to MSRs.
United Kingdom: In the United Kingdom, efforts on MSR technology are frequently done in partnership with other European nations.
High efficiency: Molten salt reactors provide greater thermal efficiency as they can function at higher temperatures [
].Safety: With passive safety features, the likelihood of nuclear accidents is significantly reduced.
Nuclear waste decrease: The thorium fuel cycle produces less and less damaging nuclear waste.
Proliferation resistance: Offers greater resistance to proliferation due to lower production of fissile material used for nuclear weapons production.
Flexibility: Can be adjusted for different fuel cycles and purposes.
Molten salt reactors demonstrate considerable potential in the field of nuclear energy. However, more research and development are needed before this technology can be commercially implemented. Specifically, additional focus should be placed on issues like material compatibility, fuel cycle, and safety.
In summary, molten salt reactors symbolize a promising development for the future of nuclear energy. Though there have been ups and downs throughout history, they are gaining renewed interest today and are viewed as an essential choice for a sustainable energy future.
Problem: An industrial facility uses a coal-based boiler system for generating steam. To reduce the energy costs of this facility and decrease its environmental impact, a new steam generation system using a Molten Salt Reactor (MSR) is being explored. In this situation, what can MSR offer for energy savings?
Answer: The answer varies depending on a number of factors. However, generally, the potential advantages of MSRs in energy savings include:
Increased thermal efficiency: MSRs offer improved thermal efficiency because they can operate at higher temperatures than other reactor types. This means that more electrical energy can be produced using the same amount of nuclear fuel.
Combined cycle: The high-temperature thermal energy from MSRs can be used not just for electricity generation but also for various applications such as steam production in industrial processes and seawater desalination. This enhances energy efficiency and ensures better utilization of energy resources.
Decrease of nuclear waste: MSRs generate a smaller amount of less harmful nuclear waste. This reduces waste management costs and lessens environmental impacts.
Continuous operation: MSRs can operate continuously for long periods. This reduces operational interruptions and enhances system efficiency.
Accurate energy savings estimation requires analyzing multiple variables such as the performance of the current coal-fired boiler system, the performance of the MSR, the fuel expenses of both systems, running costs, and the volume of steam generated must be examined thoroughly (Table 1).
Existing system: It is stated that a coal-fired boiler system works at 70% thermal efficiency.
MSR System: It is stated that the MSR system works at 90% thermal efficiency.
Yearly Fuel Use: 10,000 tons of coal are used every year in the existing system.
Fuel Price: It is stated that coal costs 150 TL per ton and nuclear fuel costs 250 TL per ton (based on energy content).
Based on this case, with the MSR system;
Current coal fuel system: 10,000 tons x 150 TL/ton = 1.500.000 TL/year.
MSR System (30% Fuel Reduction): 7.000 tons x 250 TL/ton = 1.750.000 TL/year.
Note: The higher unit cost of the thorium is balanced with reduced fuel consumption and lower waste management costs, but the initial investment costs of MSR are higher.
System scale: Calculations are based on an industrial facility of 1500 MWth, which is a representative for a medium -sized steam production facility in Turkey. MSR is modeled as a thorium fuel system with a compact design (for example, two truck containers) as stated in the article.
Geographical context: Analysis is contextualized for Türkiye where coal is a common industrial fuel and energy prices have been derived from local market data [
].Operational conditions: MSR operates with the melted salt fuel mixture at 600°C - 700 °C and provides high thermal yield. The coal system operates under standard industrial conditions (for example, 500°C - 600 °C vapor temperature). Environmental factors, such as ambient temperature, fixed (20 °C) were accepted.
Scope: Calculations focus on fuel -related energy savings and exclude capital costs, maintenance and decommission costs; because the aim is to show operational savings.
Rewording costs for construction and operation: The expenses related to installing and running an MSR system might be greater than those of a coal-fired system.
Effects on the environment: MSRs produce significantly fewer greenhouse gas emissions compared to coal-fired systems. This leads to lesser environmental expenses.
Independence in energy: Achieving energy independence is possible through the utilization of local nuclear fuel resources.
Table 2 offers a clear comparison by supporting quantitative claims and emphasizes that despite the higher unit fuel cost of MSR, it provides long -term savings potential with lower fuel consumption and reduced waste management costs, but requires higher initial investment costs.
Table 3, emphasizes the advantages of fast spectrum MSRs with thorium fuel in terms of efficiency and waste reduction, offering a systematic comparison on design options and fuel efficiency.
The examination shows that the adoption of an MSR system can result in notable energy savings and ecological advantages when compared to a conventional coal-fired boiler system. Key Findings:
MSRs, because of their improved efficiency, can significantly reduce fuel consumption, which results in reduced operational expenses. Lower Greenhouse Gas Outputs; MSRs emit considerably fewer greenhouse gas outputs, aiding in a healthier environment. Potential for energy self-sufficiency; by using local nuclear fuel resources, MSRs can improve energy security.
Nonetheless, it is vital to take into account the following aspects;
Upfront investment: The initial cost for an MSR system could be greater than that of a conventional coal-fired system.
Regulatory challenges: The rules and regulations governing nuclear energy, such as licensing and safety requirements, may influence the implementation of MSRs.
Public acceptance of nuclear technology: The acceptance of nuclear energy by the public and the risks involved is an important consideration.
In summary, although MSRs present substantial opportunities for energy savings and ecological benefits, a complete techno-economic study is required to assess their practicality in specific situations. MSRs are expected to reduce energy costs and environmental effects over time.
Fiorina C, Lathouwers D, Aufiero M, Cammi A, Guerrieri C, Leen J, Enrico M. Modelling and analysis of the MSFR transient behaviour. Ann Nucl Energy. 2014;64:485–498. Available from: https://doi.org/10.1016/j.anucene.2013.08.003
Singh V, Lish MR, Wheeler AM, Chvála O, Singh V, Lish MR, Upadhyaya BR. Dynamic modeling and performance analysis of a two-fluid molten salt breeder reactor system. Nucl Technol. 2018;1–24. Available from: https://doi.org/10.1080/00295450.2017.1416879
Zhang D, Qiu S, Su G. Development of a safety analysis code for molten salt reactors. Nucl Eng Des. 2009;239:2778–2785. Available from: https://doi.org/10.1016/j.nucengdes.2009.08.020
World Nuclear Association. Molten salt reactors [Internet]. London: World Nuclear Association; 2024 [cited 2025 Apr 23]. Available from: https://world-nuclear.org/information-library/current-and-future-generation/molten-salt-reactors
World Nuclear Association. Molten salt reactors. 2017. Available from: https://web.archive.org/web/20170518085106/http://www.world-nuclear.org/information-library/current-and-future-generation/molten-salt-reactors.aspx
Qi J, Zheng R, Wang Y, Huhe T, Ling X, Yuan H, Chen Y. Study on the pyrolysis law of tires in a molten salt heating pyrolysis reactor: experimental and CFD-DEM simulation. Energy. 2025;319:134921.
Sorensen K. Google Tech-Talk: "Why Didn't Thorium MSR Happen?". Available from: https://energyfromthorium.com/2011/12/23/techtalk-why-tmsr/
Aufiero M, Cammi A, Geoffroy O, Losa M, Luzzi L, Ricotti ME, Rouch H. Development of an OpenFOAM model for the Molten Salt Fast Reactor transient analysis. Chem Eng Sci. 2014;111:390–401. Available from: https://doi.org/10.1016/j.ces.2014.03.003
Forsberg CW. Molten-salt-cooled advanced high-temperature reactor for production of hydrogen and electricity. Nucl Technol. 2003;144:289–302.
Vijayan PK. Conceptual design of Indian molten salt breeder reactor. Pramana J Phys. 2015;85:539–554.
Türkiye Statistical Institute. Energy prices and costs in Turkey. Ankara (Turkey): TÜİK; 2023.
World Nuclear Union. The economy of nuclear energy. London: World Nuclear Union; 2023.
LeBlanc D. Erimiş tuz reaktörleri: Eski bir fikre yeni bir başlangıç. Nucl Eng Des. 2010;240(6):1644–1656
Kartal MA. Investigation and Energy Modeling of New Generation Environmentally Friendly Energy Source Thorium Fueled Molten Salt Reactors. IgMin Res. April 24, 2025; 3(4): 206-210. IgMin ID: igmin300; DOI:10.61927/igmin300; Available at: igmin.link/p300
Anyone you share the following link with will be able to read this content:
Bandırma Onyedi Eylül University, Distance Education Application and Research Center, 10200 Bandırma, Balıkesir, Turkey
Address Correspondence:
Mehmet Akif Kartal, Bandırma Onyedi Eylül University, Distance Education Application and Research Center, 10200 Bandırma, Balıkesir, Turkey, Email: [email protected]
How to cite this article:
Kartal MA. Investigation and Energy Modeling of New Generation Environmentally Friendly Energy Source Thorium Fueled Molten Salt Reactors. IgMin Res. April 24, 2025; 3(4): 206-210. IgMin ID: igmin300; DOI:10.61927/igmin300; Available at: igmin.link/p300
Copyright: © 2025 Kartal MA. This is an open access article distributed under the Creative Commons Attribution License, which permits unrestricted use, distribution, and reproduction in any medium, provided the original work is properly cited.
Fiorina C, Lathouwers D, Aufiero M, Cammi A, Guerrieri C, Leen J, Enrico M. Modelling and analysis of the MSFR transient behaviour. Ann Nucl Energy. 2014;64:485–498. Available from: https://doi.org/10.1016/j.anucene.2013.08.003
Singh V, Lish MR, Wheeler AM, Chvála O, Singh V, Lish MR, Upadhyaya BR. Dynamic modeling and performance analysis of a two-fluid molten salt breeder reactor system. Nucl Technol. 2018;1–24. Available from: https://doi.org/10.1080/00295450.2017.1416879
Zhang D, Qiu S, Su G. Development of a safety analysis code for molten salt reactors. Nucl Eng Des. 2009;239:2778–2785. Available from: https://doi.org/10.1016/j.nucengdes.2009.08.020
World Nuclear Association. Molten salt reactors [Internet]. London: World Nuclear Association; 2024 [cited 2025 Apr 23]. Available from: https://world-nuclear.org/information-library/current-and-future-generation/molten-salt-reactors
World Nuclear Association. Molten salt reactors. 2017. Available from: https://web.archive.org/web/20170518085106/http://www.world-nuclear.org/information-library/current-and-future-generation/molten-salt-reactors.aspx
Qi J, Zheng R, Wang Y, Huhe T, Ling X, Yuan H, Chen Y. Study on the pyrolysis law of tires in a molten salt heating pyrolysis reactor: experimental and CFD-DEM simulation. Energy. 2025;319:134921.
Sorensen K. Google Tech-Talk: "Why Didn't Thorium MSR Happen?". Available from: https://energyfromthorium.com/2011/12/23/techtalk-why-tmsr/
Aufiero M, Cammi A, Geoffroy O, Losa M, Luzzi L, Ricotti ME, Rouch H. Development of an OpenFOAM model for the Molten Salt Fast Reactor transient analysis. Chem Eng Sci. 2014;111:390–401. Available from: https://doi.org/10.1016/j.ces.2014.03.003
Forsberg CW. Molten-salt-cooled advanced high-temperature reactor for production of hydrogen and electricity. Nucl Technol. 2003;144:289–302.
Vijayan PK. Conceptual design of Indian molten salt breeder reactor. Pramana J Phys. 2015;85:539–554.
Türkiye Statistical Institute. Energy prices and costs in Turkey. Ankara (Turkey): TÜİK; 2023.
World Nuclear Union. The economy of nuclear energy. London: World Nuclear Union; 2023.
LeBlanc D. Erimiş tuz reaktörleri: Eski bir fikre yeni bir başlangıç. Nucl Eng Des. 2010;240(6):1644–1656